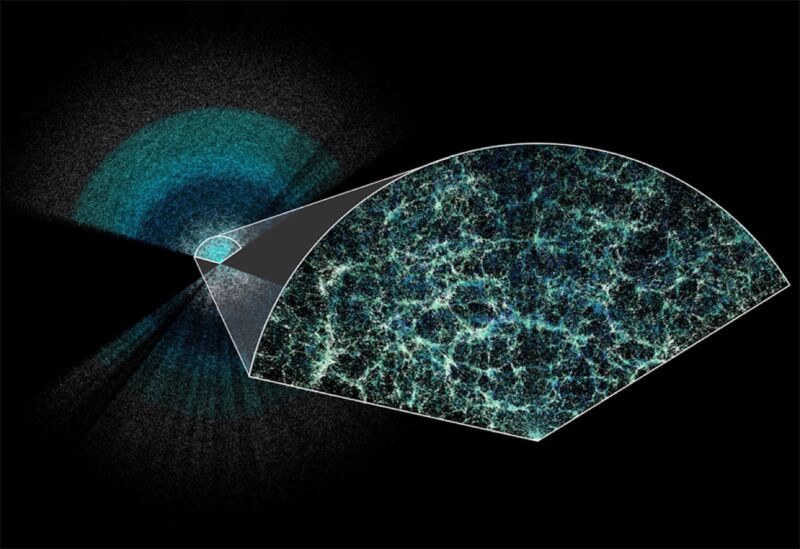
An international collaboration of scientists has created the largest 3D map of our universe to date based on the first results from the Dark Energy Spectroscopic Instrument (DESI). It's an impressive achievement, with more to come, but the most significant finding stems from the collaboration's new measurements of dark energy. Those results roughly agree with the current prevailing theoretical model for dark energy, in which dark energy is constant over time. But there are some tantalizing hints that it could vary over time instead, which would call for some changes to that prevailing model.
Granted, those hints are still below the necessary threshold to claim discovery and hence fall under the rubric of "huge, if true." We'll have to wait for more data from DESI's continuing measurements to see if they hold up. In the meantime, multiple papers delving into the technical details behind these first results have been posted to the arXiv, and there will be several talks presented at a meeting of the American Physical Society being held this week in Sacramento, California, as well as at Rencontres de Moriond in Italy.
“Our results show some interesting deviations from the standard model of the universe that could indicate that dark energy is evolving over time,” said Mustapha Ishak-Boushaki, a physicist at the University of Texas, Dallas, and a member of the DESI collaboration. “The more data we collect, the better equipped we will be to determine whether this finding holds. With more data, we might identify different explanations for the result we observe or confirm it. If it persists, such a result will shed some light on what is causing cosmic acceleration and provide a huge step in understanding the evolution of our universe.”
AdvertisementHitting the accelerator
What is the universe made of? The current consensus is that ordinary matter accounts for a mere 4 percent of all matter in the universe, while dark matter accounts for another 23 percent. Dark energy accounts for the remaining 73 percent, and that dark energy is the driving force behind the universe's accelerating expansion rate.
There was a time when scientists believed that the Universe was static, but that changed with Albert Einstein's general theory of relativity. Alexander Friedmann published a set of equations in 1922 showing that the Universe might actually be expanding, with Georges Lemaitre later making an independent derivation to arrive at that same conclusion. Edwin Hubble confirmed this expansion with observational data in 1929. Prior to this, Einstein had been trying to modify general relativity by adding a cosmological constant to get a static universe from his theory; after Hubble's discovery, legend has it, he referred to that effort as his biggest blunder.
Scientists expected that if the universe were still expanding, the attractive force of gravity would eventually slow down the rate of expansion. But in 1998, two separate teams of physicists measured the change in the universe’s expansion rate, using distant supernovae as mileposts. When Hubble made his 1929 measurements, the farthest red-shifted galaxies were roughly 6 million light-years away. If expansion was now slowing under the influence of gravity, supernovae in those distant galaxies should appear brighter and closer than their red shifts would suggest.
Instead, just the opposite was true. At high red shifts, the most distant supernovae are dimmer than they would be if the universe were slowing down. Instead of gradually slowing down, the expansion of the universe is speeding up. The leaders of those two teams went on to win the 2011 Nobel Prize in Physics. Those 1998 results have since been corroborated by improved supernovae measurements, as well as indirect measurements of the cosmic microwave background (CMB), gravitational lensing, and the large-scale structure of the cosmos.
AdvertisementIf dark matter gives rise to the gravity that holds the universe together, then dark energy is the counter-force pushing the universe apart. Very early in the universe’s existence, dark matter dominated. Everything was closer together, so its density was higher than that of the dark energy, and its gravitational pull was stronger so the early galaxies could form. But as the universe continued to expand, the dark matter density, and hence the gravitational pull, decreased until it was less than that of the dark energy. So instead of the expected slowdown in the expansion rate, the now-dominant dark energy began pushing the universe apart at ever-faster rates.
Einstein’s cosmological constant (lambda) implied the existence of a repulsive form of gravity. Quantum physics holds that even the emptiest vacuum is teeming with energy in the form of “virtual” particles that wink in and out of existence, flying apart and coming together in an intricate quantum dance. This roiling sea of virtual particles could give rise to dark energy, giving the universe a little extra push so that it can continue accelerating. The problem is that the quantum vacuum contains too much energy: roughly 10120 times too much.
So the universe should be accelerating much faster than it is if the dark energy is, essentially, the cosmological constant. Still, all the observations to date indicate that it's constant. The best theoretical fit is known as the Lambda CDM model, which incorporates both a weakly interacting cold dark matter and dark energy. One alternative theory proposes that the universe may be filled with a fluctuating form of dark energy dubbed “quintessence.” There are also several other alternative models that assume the density of dark energy has varied over the history of the universe.
Here's where the potentially exciting initial findings of DESI enter the chat.
The next generation
In its earliest days, the universe was a hot, dense soup of subatomic particles, including hydrogen and helium nuclei, aka baryons. Tiny fluctuations created a rippling pattern through that early ionized plasma, which froze into a three-dimensional place as the universe expanded and cooled. Those ripples, or bubbles, are known as baryon acoustic oscillations (BAO). It's possible to use BAOs as a kind of cosmic ruler to investigate the effects of dark energy over the history of the universe.
That's what DESI was designed to do: take precise measurements of the apparent size of these bubbles (both near and far) to determine distances to galaxies and quasars over 11 billion years. That data can then be sliced into chunks to determine how fast the universe was expanding at each point of time in the past, the better to model how dark energy was affecting that expansion. "What this is doing is creating the largest 3D map of the universe to tell us about dark energy," Nathalie Palanque-Delabrouille, a physicist at Lawrence Berkeley Laboratory and co-spokesperson for DESI, told Ars.
The DESI collaboration released the first batch of data (80 terabytes) last June, taken during the first two months, aka the "survey validation" phase, in order to verify that the instrument was working as expected with regard to its measurements. That "One Percent Survey" was sufficient to create an initial 3D map of 700,000 galaxies and quasars and a nifty fly-through video. DESI will ultimately map 3 million quasars and 37 million galaxies by the end of its planned five-year run. Scientists were also able to use that dataset to find evidence of a mass migration of stars into the Andromeda galaxy.
AdvertisementThese latest results are based on analysis of a full year's worth of data taken from seven different slices of cosmic time and include 450,000 quasars, the largest ever collected, with a record-setting precision of the most distant epoch (between 8 to 11 billion years back) of 0.82 percent. While there was basic agreement with the Lamba CDM model, when those first-year results were combined with data from other studies (involving the CMB or Type Ia supernovae), some subtle differences cropped up. Essentially, the dark energy might be getting weaker.
In terms of confidence, those results amount to a 2.6-sigma level for the DESI's data combined with CMB datasets. That confidence level remains or even grows when adding the supernovae data: 2.5-sigma, 3.5-sigma, or 3.9-sigma levels, depending on which particular supernova datasets are used. That qualifies as an intriguing hint, below the threshold for discovery; more data will eventually either confirm those discrepancies or they will disappear.
Nonetheless, “It may be the first real clue we have gotten about the nature of dark energy in 25 years,” Adam Reiss, a physicist at Johns Hopkins University who shared the 2011 Nobel Prize in Physics, told the New York Times. “This result is very interesting and we should take it seriously. Otherwise why else do we do these experiments?”
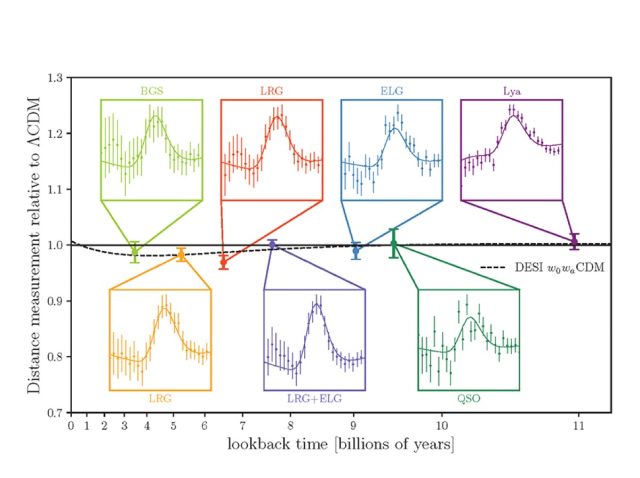
"It's not yet a clear confirmation, but the best fit is actually with a time-varying dark energy," said Palanque-Delabrouille of the results. "What's interesting is that it's consistent over the first three points. The dashed curve [see graph above] is our best fit, and that corresponds to a model where dark energy is not a simple constant nor a simple Lambda CDM dark energy but a dark energy component that would vary with time. For the past 25 years, all the measurements we had actually made it sound like the simple solution might have been the good one."
Why it matters
The question of whether the dark energy density varies over time or remains constant is central to determining how our universe will end. If it's constant, the acceleration will continue indefinitely, and matter will grow farther and farther apart. Within a hundred billion years, we will only be able to see a few hundred galaxies, compared to the hundreds of billions we can see today—i.e., a slow, lingering death for the universe. If the dark energy varies, the fate of the cosmos depends on whether that density decreases or increases over time. If the dark energy decreases, expansion could slow enough for the universe to recollapse in a Big Crunch. And if the dark energy increases its expansion rate over time, it could rip apart every galaxy, star, and atom within 100 billion years, a scenario dubbed “the Big Rip.”
DESI's 3D large-structure map contains information beyond baryonic acoustic oscillations. For instance, there could be future implications for the ongoing debate over the value of the Hubble constant. There are basically three methods scientists use to measure the Hubble Constant: looking at nearby objects to see how fast they are moving, gravitational waves produced by colliding black holes or neutron stars, and measuring tiny deviations in the CMB. However, the various methods have come up with different values.
Advertisement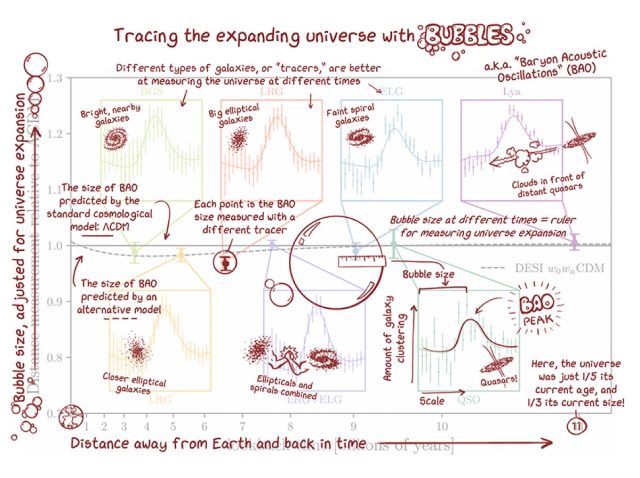
In short, we've measured it using information in the cosmic microwave background extrapolated to today and gotten one value. And we've measured it using the apparent distance to objects in the present-day Universe and gotten a value that differs by about 10 percent. As far as anyone can tell, there's nothing wrong with either measurement, and there's no obvious way to get them to agree. DESI provides yet another valuable independent dataset. DESI's preliminary results indicate a value "maybe slightly larger" than CMB measurements but still roughly "in the same ballpark," according to Palanque-Delabrouille.
DESI's map is also sensitive to neutrino masses, another point of debate among physicists. The Lambda-CDM model puts tight restrictions on the upper limit of neutrino masses. That could change if dark energy turns out to be varying rather than constant: the upper boundary would increase slightly, per Palanque-Delabrouille. "It is still much more stringent than anything we would get from direct measurements of neutrino mass, but we still can't tell what the masses are," she said. "We're getting tighter and tighter upper bounds, but they are still very sensitive to the assumptions we make [in our models]. At some point we would like to see a peak [in the data], not just an upper bound."